Nuclear fusion is a natural process, integral to all life on earth.
The sun, a huge plasma ball containing 99.8% of the total mass of our Solar System, has a fusion fire burning constantly at its core – the energy created in these fusion reactions is what heats and lights the earth.
Research into nuclear fusion aims to develop power plants that, like the sun, can generate vast amounts of climate friendly energy: 1 gram of fusion fuel could generate 90,000 kWh, the equivalent combustion heat of 11 tonnes of coal.¹
One of the technologies being explored in the quest for real-world fusion power is the ‘Stellarator’ – a machine that uses magnetic fields to confine plasma in a torus (the geometrical term for a donut shape.) Using these magnetic fields, research scientists are able to control the plasma particles and create the right conditions for fusion reactions. Whilst Stellarators could provide a technically simpler solution than other experimental methods of nuclear fusion energy (i.e., the Tokamak design) as they require less injected power to sustain the plasma, there is increased complexity to the plasma theory, particularly when it comes to the magnetic field coils.
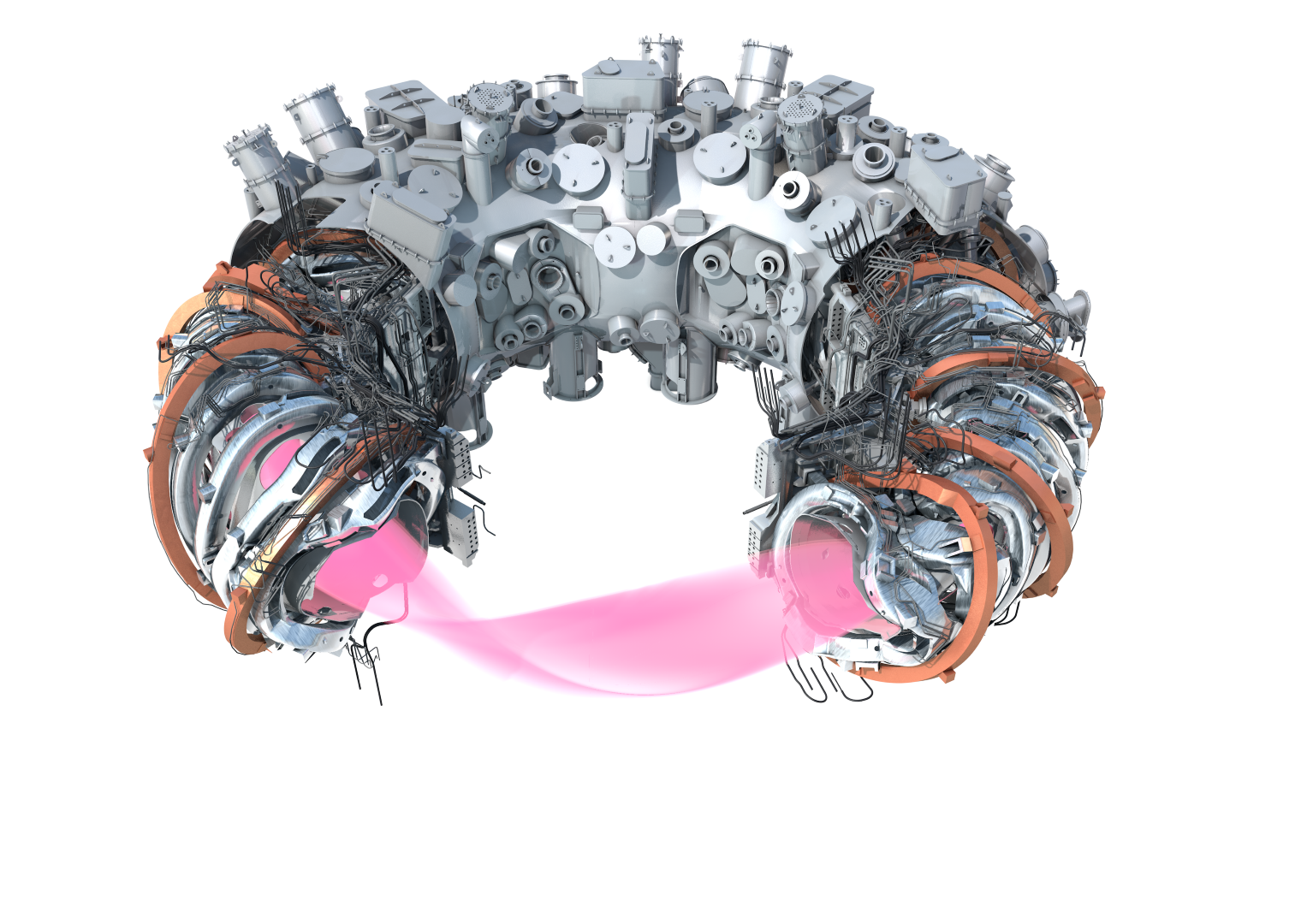
Plasma vessel, superconducting stellarator magnet coils, planar magnet coils, support structure and cryostat of the Wendelstein 7-X fusion device. (Graphic: IPP)
The Max-Planck-Institute for Plasma Physics (IPP) concentrates its research on seven experimental nuclear fusion projects. Of these projects, Wendelstein 7-X based at Greifswald in Germany, is the world’s largest Stellarator and its objective is to investigate the suitability of the design for a working power plant. A ring of 50 superconducting magnetic coils makes up a confinement cage; the shape of each coil determined by complex calculations, optimised to reach the quality level of plasma confinement available in Tokamak-type facilities.
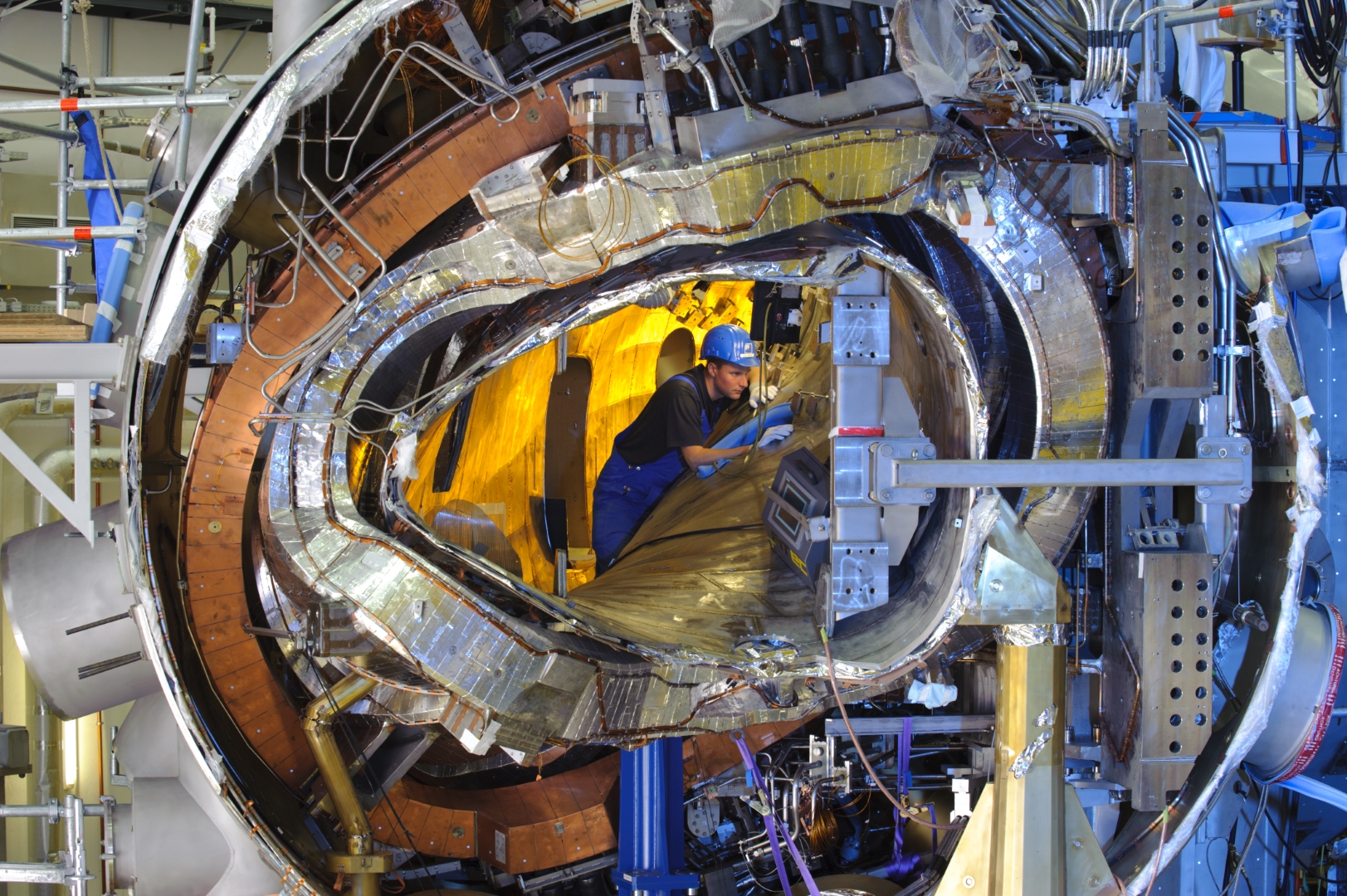
View inside: Visible are the plasma vessel, a magnet coil, the outer casing, numerous coolant ducts and power leads (photo: IPP)
Whilst fusion fuels are cheap and readily available (seawater contains Deuterium in almost inexhaustible quantities, Tritium can be formed from the abundantly available Lithium²), it is the exacting conditions necessary to create plasma that present a major challenge when designing nuclear fusion research sites of either design. Increasing temperature transforms materials from solid to liquid and then to gas. Further (and extreme) increases in temperature are then required to reach the ‘4th state of matter’ – the electrically conductive, ionized gas called plasma.
On Wendelstein 7-X there are around 50 scientific subsystems designed to measure certain parameters of the plasma and its surrounding environment – temperature, density, energy content, currents in the plasma, impurities, etc. The extreme conditions – fusion fuel is heated up to 100 million degrees Celsius to reach the plasma state – call for special measuring methods to investigate these diagnostics.
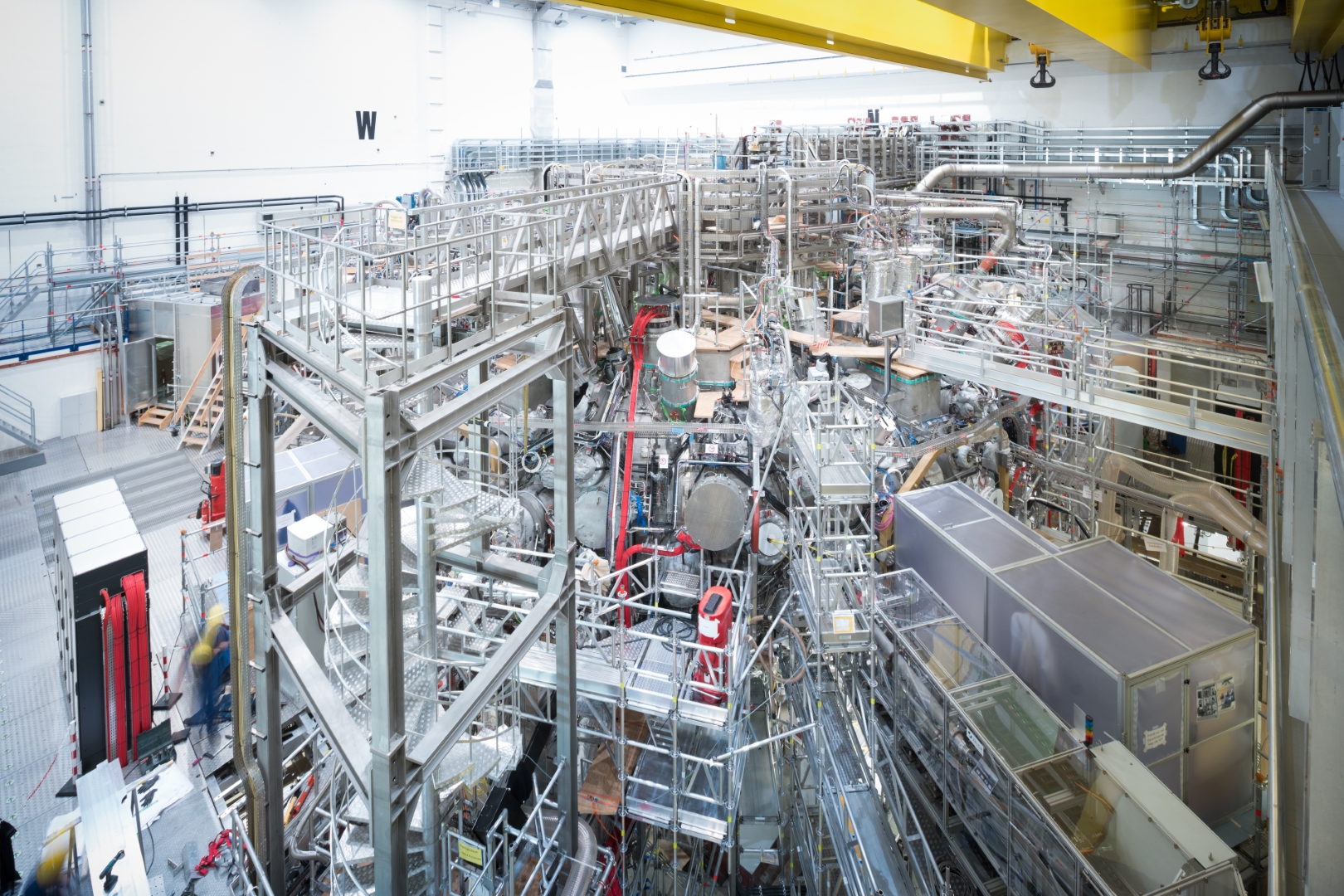
View of Wendelstein 7-X with its numerous diagnostics (photo: IPP, Jan Michael Hosan)
Whilst much of the measurement hardware utilised by IPP is designed for direct readout, strict access restrictions during operation make it impossible for scientists to view HMIs or screens, and so all of the diagnostics must be remotely monitored. Brainboxes Ethernet to Serial (ES) devices are used to send critical data from sensors installed in the diagnostic ports and the connected serial-bus-only controllers, over the network to be remotely monitored on the control PC stationed a safe distance away.
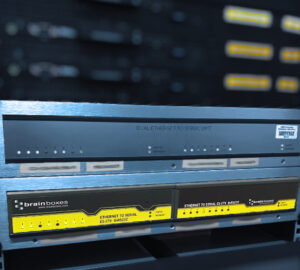
Brainboxes Ethernet to Serial devices in-situ
Even with the pandemic unavoidably delaying progress, the Wendelstein 7-X experiment has now completed its 20-year construction program, going into commissioning operation with full scientific operation planned to begin this Autumn 2022. Bridging the gap between the diagnostic ports and the control PC with Ethernet to Serial devices, saved time and allowed IPP engineers to build the diagnostic quickly and with fewer hardware requirements.
With the time-sensitive need to establish environmentally-sound, sustainable sources of energy, and the fact that questions about nuclear fusion can’t be answered by theoretical means; clarifying it experimentally with functioning sites like IPP at Greifswald means that fusion power plants could start supplying real-world electricity as early as the second half of this century.
-
ES-279
$658.90 Add to cart -
ES-522
$408.10 Add to cart
¹ Max Planck Institute for Plasma Physics